Camera Lens Anti-Reflection Coatings: Magic Explained
There’s more science and history that goes into camera lens coatings than you could imagine. Without the invention of anti-reflection lens coatings, modern lenses couldn’t exist.
With nothing added to the surface of a glass lens, about 4% of the light hitting it gets reflected back and is lost. A camera lens with only a single element has two air-glass surfaces, so the reflection losses double. Modern lenses often have upwards of 20 elements in them, so un-coated surfaces would result in huge light losses.
Much of the light that gets reflected off of lens surfaces will bounce around inside the lens and end up fogging your image with horrible flare.
In 1935, the Ukranian physicist Alexander Smakula of the Zeiss company invented the world’s first anti-reflection coating. This invention was so important that it became a German military secret. The Allies only discovered this secret in early World War II, and so lens anti-reflection coating knowledge quickly spread worldwide.
You may have heard of Zeiss T* optics. The “T” is short for “transparency”, and alludes to the anti-reflection coatings used in their lenses to yield superior optical transparency.
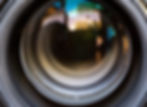
Light reflecting off of a coated lens
Notice above that there are a pair of reflections shown. A bush in the upper reflection looks green, while the same bush reflection looks yellow as the light bounces off of a different lens surface with a different coating on it.
Somehow, the lens is reflecting different colors of light off of different lens element surfaces. Read further to get some insight into how a lens maker can cause this to happen.
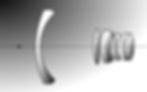
Typical camera lens design with several glass elements
A typical camera lens with six elements is shown above. Each air-glass surface gives more opportunity for light to reflect back, instead of being transmitted through the whole lens. Picture courtesy of Wikipedia.

Light wave reflecting off of a coated lens
There’s a lot to explain in the picture shown above, so here goes.
The Greek symbol “lambda” is λ. This symbol is commonly used to represent the wavelength of light. For camera lenses, the most important light wavelength to worry about is green (the same reason that there are twice as many green pixels in camera sensors as there are red or blue pixels). Green light wavelength is about 500 billionths of a meter.
The letter η shown above represents the “index of refraction”. The index of refraction indicates how much power a substance has to bend light. The η₀ above represents the index of refraction of air, while η₁ is the index of refraction of the lens coating, and ηₛ is the index of refraction of the lens glass itself. Light actually slows down when traveling through a substance with a high index of refraction (it only moves “at the speed of light” while in a vacuum, whose index of refraction value is 1.0).
The R₁ above is the light wave that gets reflected off of the lens coating. The R₂ above is the light wave that is reflected off of the lens glass element surface. These reflected light waves are drawn in orange. The coating material is shown in darker blue, while the lens element glass is shown in light blue.
The “T” above is the transmitted light wave that has survived the journey through the lens coating and the surface of the lens glass. The drawing above is slightly inaccurate, because the transmitted light wave is actually "bent" and exits the lens in a different direction than when it entered the lens.
Notice that the lens coating thickness shown above is a quarter of the light wavelength (¼λ). This is no accident. The distance traveled by the reflected light wave R₂ is a half-wavelength farther than the light wave R₁ that reflects off of the lens coating. The extra half wavelength of travel happens because it goes a quarter wavelength through the coating, and then reflects back another quarter wavelength to exit the coating.
When the pair of reflected light waves R₁ and R₂ combine, their wave crests and troughs now align. This alignment difference causes the waves to cancel each other and “disappear”, which is called “destructive interference” by physicists.
Since the reflected light waves (the green ones, at least) cancel each other out, all of the incoming light energy gets transmitted through the glass lens element instead of having some (about 4%) being lost due to reflection!
Incredibly, by forcing an extra reflection off of the lens, you end up not losing any light, and it all gets transmitted through the lens! This is the opposite of what your “common sense” would tell you would happen when forcing extra reflections. Now you can start to appreciate the genius of Alexander Smakula (he went on to teach and do research at MIT in the United States).
The discussion above is only “theoretical”, however. The lens coating index of refraction η₁ should ideally be half of the value in between that of air η₀ and the lens glass ηₛ. The index of refraction of typical lens glass is about 1.5, while the index of refraction of air is 1.0003. The ideal lens coating index of refraction would then be about 1.25.
Reality rears its ugly head, though. The substance “magnesium fluoride” has an index of refraction of 1.38. It is transparent and can be applied after heating in an oven (to form a vapor) and deposit a thin coating onto lenses. This coating thickness can be carefully controlled, so they can put down a quarter-wavelength- thick layer. Magnesium fluoride is tough enough to withstand the rigors of daily use and lens cleaning. This type of lens coating isn't the only substance used by lens manufacturers, but it's probably the most common.
Imagine if you had to accurately apply 125 billionths of a meter of something onto glass. And people expect this to get done for cheap. Good luck.
They haven’t yet found a substance that is closer to the ideal index of refraction of 1.25 that has the mechanical and optical properties needed for a lens coating. At least nobody is confessing to knowledge of this desirable substance.
The net result is that they can make single-coated lenses that reduce reflections down to about 1%, versus the theoretical 0%.
Light waves arrive with different colors (therefore different wavelengths). This means that the coating thickness optimized for green light isn’t very efficient in reducing these other reflections. Light waves also strike the lens at different angles, and therefore they don’t travel precisely one-half-wavelength farther to cause the perfect reflection-canceling behavior.
So guess what? Lenses now get “multi-coating”. These multiple coating layers on lens surfaces cause yet more reflections. The outer coating layers (with slightly lower index of refraction values) can further reduce (via reflection destructive interference) light losses.
If each layer of a coating on a lens surface is a different thickness, then it becomes possible to stop the losses of different colors of light.
Just like the Germans before the War did, lens makers aren’t divulging their secrets of just exactly how they’re making lens multi-coatings that enable near-perfect light transmission.
Thanks, Alexander.